Dealing with an Uncertain Future: Direct Reduction in the Hydrogen Economy
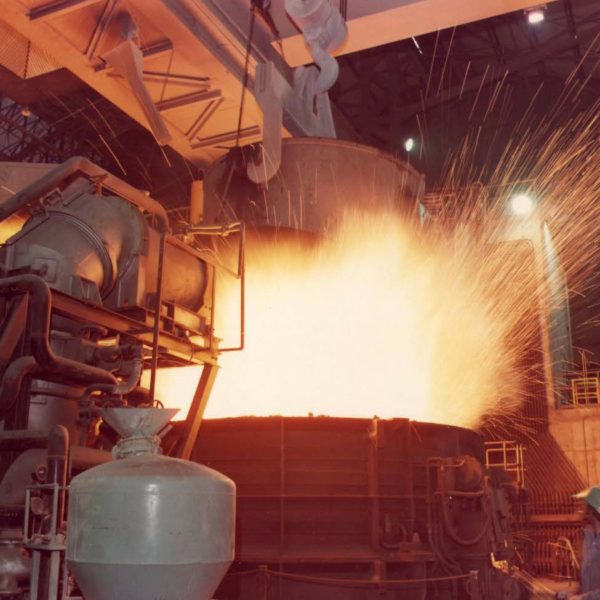
The steel industry is being faced with exciting opportunities: new fuels, the mitigation of greenhouse gas (GHG) emissions, new technologies, and new raw materials are all leading to discussions and ideas that would not have been possible even a few short years ago.
Unfortunately, new opportunities also cause new uncertainties which can complicate decisions that have long investment timelines. The direct reduction of iron ore using the MIDREX® Process can help mitigate these uncertainties by providing unparalleled flexibility for raw materials, fuels, and product offerings. This article will discuss the evolving market opportunities and uncertainties and show how direct reduction technology offerings can help meet these future requirements.
GROWTH OF DIRECT REDUCED IRON (DRI)
In 2020, the International Energy Agency (IEA) projected the growth of several ironmaking process routes in their Energy Technology Perspectives. The projections are summarized in Table I.
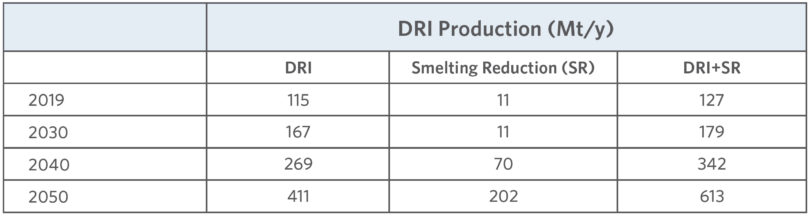
TABLE I.
Summary of ironmaking routes from the IEA Energy Technology Perspectives 2020
The IEA projects that DRI could grow to over 400 Mt/y production by 2050. That would represent rapid growth of iron production through the direct reduction route considering that the total production in 2019 is shown as 115 Mt/y. The same IEA projection shows a decline in the conventional blast furnace (BF) route for ironmaking. The projection would be a major shift away from the near monopoly of the BF route for ironmaking. The future production by process is unknowable; however, the market and technology uncertainties driving the projections can be analyzed.
One of the major factors is the increased attention being given to the environmental impacts of ironmaking. Overall ironmaking contributes between 7% to 9% of the total worldwide green-house gas emissions (also referred to as carbon mitigation or avoidance). The discussion on how to reduce the emissions from ironmaking is driving much of the uncertainty related to projections for the various ironmaking routes.
The following discussion will examine the technological impacts of various carbon mitigation or avoidance schemes for natural gas or hydrogen-based direct reduction technologies.
WHY IS HYDROGEN-BASED DRI GAINING INTEREST?
Natural gas-based direct reduction technologies have an intrinsic reduction in carbon dioxide intensity compared to the coal-based routes. The overall governing reactions are shown as follows:
Equations (1) and (2) are the reforming reactions for CH4. Equation (3) is the reduction of hematite to metallic iron by carbon, and Equations (4) and (5) are the reduction of hematite to metallic iron by hydrogen and carbon monoxide, respectively. Using the respective molecular weights, the theoretical minimum carbon dioxide intensities for Equations (3), (4), and (5) can be calculated, as shown in Table II.
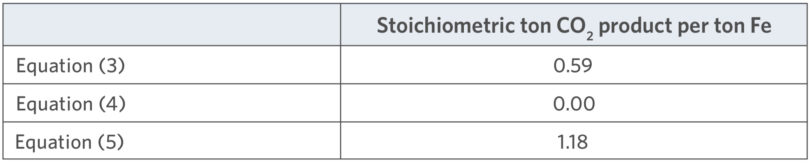
TABLE II.
Stoichiometric CO2 Intensity for Selected Reactions
Equation (4) shows that carbon emissions can be completely avoided by reducing the iron with hydrogen. Equation (3) results in a lower stoichiometric emission of CO2 than Equation (5) simply because the CO in Equation (5) already has an oxy-gen atom bound to the C. Conventional direct reduction can operate across a wide range of H2/CO ratios. The effect of H2/CO ratio on the carbon dioxide intensity can be seen in Figure 1.
Figure 1 covers a range of industrially relevant H2/CO ratios. The lower range of 0.5 H2/CO ratio is observed when high CO and CO2 containing gas is supplied to the direct reduction facility. For example, COREX® plants paired with the MIDREX Process operate with an H2/CO ratio in this range. The higher end of the range is observed when steam methane reformers (SRM) provide the syngas to the direct reduction plant. H2/CO ratios even higher than 3 are possible; however, Figure 1 shows that the curve will continue to approach zero CO2 Intensity asymptotically as the H2/CO ratio increases.
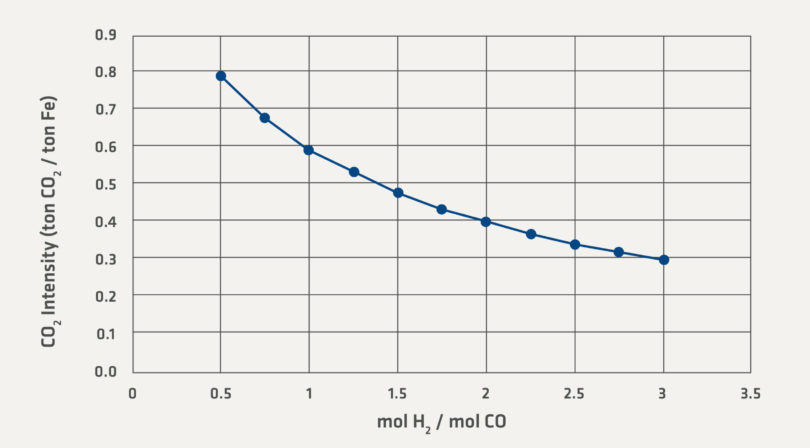
FIGURE 1.
Stoichiometric CO2 for a Range of Reducing Gas Compositions
The CO2 intensity has been treated from a pure chemical reaction point-of-view up to this point. Figure 1 also shows the importance of the reforming techniques and gas recycling systems in natural gas-based direct reduction facilities. All of the major natural gas-based direct reduction technologies operate with a method to process and recycle the spent reducing gases back to the shaft furnace. Because of the recycle loop and other processing requirements, the actual emissions calculated here do not reflect the actual observed CO2 emissions reported from the industrial facilities. In the industrial application, the CO2 emission can be approximated from the natural gas consumption. Generally, direct reduction facilities operate with emissions of approximately 0.5 ton CO2/ton DRI (typical DRI produced from DR-grade pellets contains around 90% total Fe).
In the commercial direct reduction facility, the recycled gas does not contain pure reductants (i.e., hydrogen and carbon monoxide). Because of the gas processing and recycling loops the actual gas compositions have to be calculated.
Figure 2 is calculated to illustrate the sensitivity of the gas compositions for H2 and CO for a theoretical reducing gas. In Figure 2, the ratio of H2 and CO is held high enough compared to the oxidizing H2O and CO2 to produce DRI of industrially relevant reduction degree. There is also a small percent-age of N2 and CH4 which is not shown in the graph and is the reason why the H2 and CO compositions sum to 87 vol%.
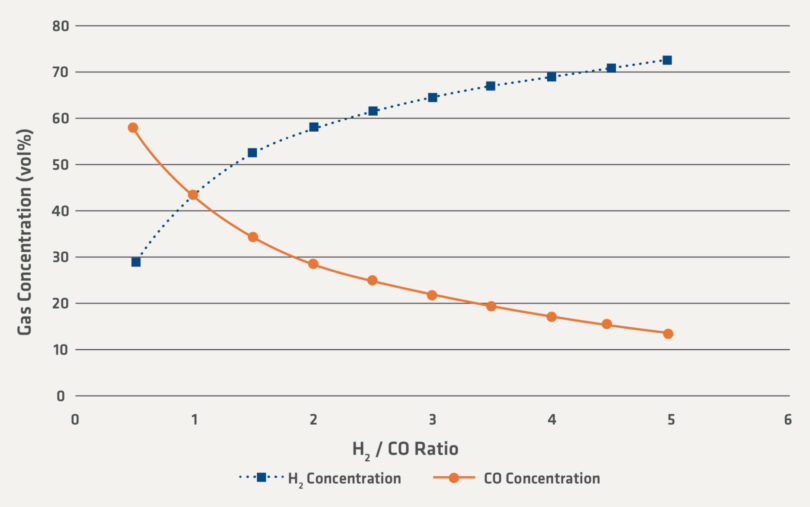
FIGURE 2.
Illustrative Gas Concentration of H2 and CO for a Range of H2/CO Ratios
One of the technological options for carbon avoidance is the substitution of green hydrogen for fossil-fuel derived hydrogen. Green hydrogen is assumed to be hydrogen that is produced with no associated CO2 emission.
When green hydrogen is introduced into the direct reduction facility, the concentration of hydrogen in the recycling gas will increase.
One of the uncertainties that the industry faces are how the direct reduction processes will be changed by increased hydrogen in the recycling gas loops. Direct reduction facilities have already operated commercially with H2/CO ratios of 3 or higher. Using the conservative basis in Figure 2, industrial direct reduction facilities have operated above 65 vol% H2 and in some cases above 70 vol% H2 in the recirculating gas loops.
The desire to directly avoid CO2 emissions by substituting green hydrogen for CH4 is driving much of the interest in direct reduction along with the IEA forecast for the growth of ironmaking by routes other than the blast furnace.
SENSITIVITY STUDY
The preceding section addressed the driving force for why hydrogen-based direct reduction is gaining interest. In this section we will examine some of the implications of increasing hydrogen reduction for the heat balance of a direct reduction shaft furnace. For the purpose of this discussion, several theoretical cases have been setup according to the conditions illustrated in Figure 3.
In Figure 3, hematite is introduced at 25 °C and calculated to adiabatically react fully to metallic iron with a fixed exit temperature of 840°C, which is industrially relevant, as it is within the range of temperatures that are generally encountered in countercurrent direct reduction. The heat balances are calculated with several inlet gas sensitivities, which are shown in Table III. The exit gas temperature and composition are calculated according to the inlet gas com-positions. Note that the calculation is purely stoichiometric and does not consider the kinetics or any limiting chemical equilibrium. The conditions for the sensitivity and the graphs showing the results are presented on the following page.
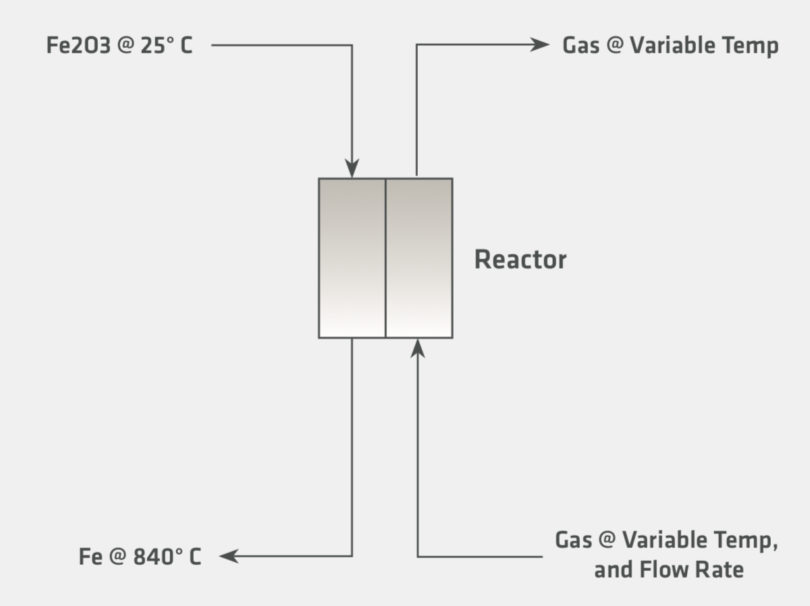
FIGURE 3.
Graphic Representation for the Thermodynamic Calculation
Figures 4 and 5 illustrate the differences in the heat requirements for reduction by H2 and reduction by CO. In the calculation, 100% H2 requires significantly higher flow rates compared to reduction by CO. However, even for the 100% H2 case, it is noteworthy that that inlet gas temperature and flow rates for the sensitivity are within the range of industrially observed operating conditions.
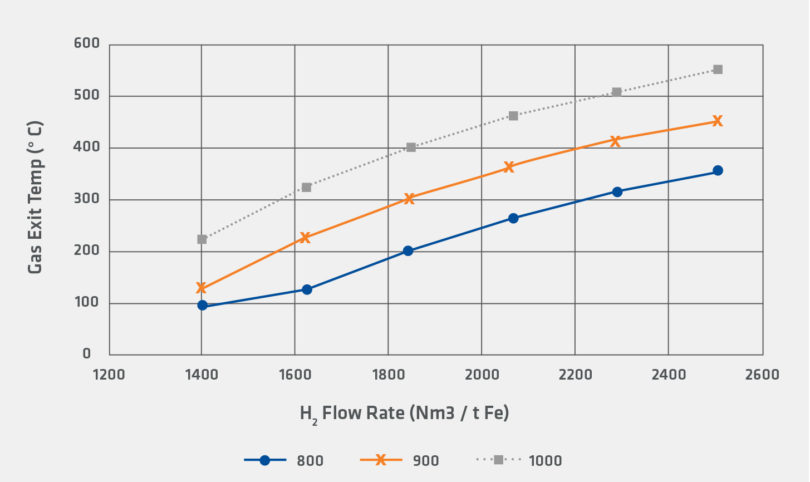
FIGURE 4.
Sensitivity for Gas Exit Temperature for H2 Reducing Gas
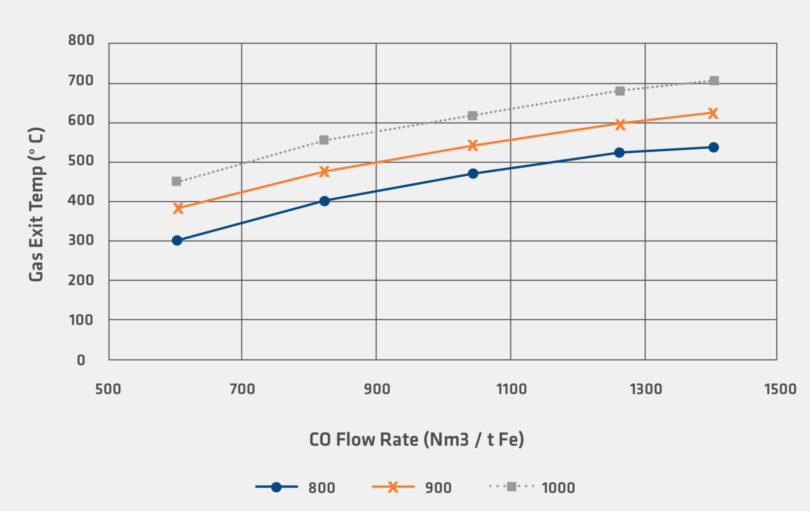
FIGURE 5.
Sensitivity for Gas Exit Temperature for CO Reducing Gas
IMPACTS FOR DIRECT REDUCTION
The purpose of the direct reduction plant is to produce DRI of sufficient metallization and temperature for the downstream users. Previously, cold direct reduced iron (CDRI) was discharged from the furnace at near ambient temperature. However, hot direct reduced iron (HDRI) and hot briquetted iron (HBI) are now the most important DRI products due to their improved energy efficiency or superior material handling properties. The hot discharge application in direct reduction has the additional operational constraint that high material discharge temperature is desirable and must be maintained. The rest of the discussion will focus only on HDRI or HBI applications.
The calculations shown previously chose the DRI temperature specifically to match the conditions required for HDRI or HBI production. These conditions give rise to the costs and benefits of avoiding carbon dioxide emissions versus the need to maintain product quality and plant productivity.
It is clear that increasing use of green hydrogen can be employed to directly avoid the emission of carbon dioxide from the direct reduction facility. However, as shown in the preceding sensitivity analysis, the hydrogen reduction reaction is more endothermic than the corresponding carbon monoxide reduction reaction. Figure 5 also indicates that the required process change to offset this higher endothermic load is to increase the flow of recycled gas to the shaft furnace.
Since the shaft furnace is an adiabatic countercurrent moving bed reactor, it is clear that the recycled gas that is introduced to the shaft furnace bustle must carry all the energy needed to satisfy the shaft furnace heat balance requirements. The main process change to offset the addition of green hydrogen to the direct reduction process is to simply increase the flow rate of gas that is circulated to the shaft furnace bustle.
The overall advantage for direct reduction is the proven operation that has significantly reduced direct CO2 emissions when compared to the conventional blast furnace route. Although the supply and economics of green hydrogen is currently uncertain, it seems likely that continuing environmental and greenhouse gas emission concerns will drive interest in this fuel as a path toward carbon dioxide emission avoidance. The direct reduction plant has the option to begin transitioning to green hydrogen as it becomes available on the market. The primary modification that can be envisioned is that at some point, the recycle gas compressors capacity may need to be upgraded. The impact and discussion on other unit operations becomes technology and case specific.
CONCLUSIONS
Direct reduction is experiencing a surge of interest due to changing market pressures for environmental concerns, especially greenhouse gas emissions. The advantage of avoiding carbon dioxide emissions directly through the use of green hydrogen is complicated due to uncertainty as to when green hydrogen will be available at the scale needed for ironmaking. Direct reduction has the technological capability to operate with up to 100% H2 as the reductant. Just as importantly, direct reduction is flexible in the fuels that it can use.
Direct reduction facilities have already demonstrated commercial operation across a wide range of process conditions. In particular, for green hydrogen as fuel, direction reduction has a demonstrated track record for H2/CO ratios of 0.5 to 3.0 or higher. Because of the gas recycling systems, the direct reduction plant can be designed for high energy efficiency across a wide range of fuels. This inherent process flexibility is critically important when the fuel supplies of the future are not certain.
Note: This article is based on a paper titled, “Direct Reduction Dealing with an Uncertain Future” by Todd Astoria, which was presented at the AISTech 2022 Exposition & Conference in Pittsburgh, PA
References:
1. IEA (2020), Iron and Steel Technology Roadmap, IEA, Paris
2. “World Direct Reduction Statistics 2020” compiled by Midrex reports the combined world DRI production for 2019 as 108.1 Mt
3. Production statistics published by the World Steel Association